Geochemistry, Mineralogy, Petrology, and Volcanology
The geochemistry, mineralogy, petrology, and volcanology group leads integrated field, laboratory and experimental research to unravel several challenging sets of problems in igneous and metamorphic petrology, economic geology, geochemistry, hydrology, and volcanology. Topics include:
Hydrothermal systems
We aim to understand how hydrothermal fluids mobilize and concentrate base metals and rare earth elements in the Earth’s crust. To achieve that, we characterize the chemical composition, temperature, and pressure of hydrothermal fluids through analyses of fluid inclusions and minerals. With this information, we can model mechanisms by which elements are leached from source rocks and eventually precipitated in mineral deposits.
Timescales of magmatic processes
Understanding the timescales of magmatic processes is essential to understanding pre-eruptive changes and syn-eruptive processes that impact volcanic eruption behavior. One method by which this is achieved is through zircon “petrochronology”, where sections of minerals are geochemically characterized and linked to crystallization ages, usually determined by U/Pb dating methods. We use these observations, combined with numerical modeling, to understand the genesis and evolution of magmas and their relationship to crustal development. Another method by which this is achieved is through diffusion chronometry. This technique leverages concentration gradients in crystals and glass that form during system perturbations (e.g., magma degassing or mixing) to calculate time between the perturbation and magma quench. Diffusion chronometry can track a range of timescales, from millions of years to seconds, depending on the diffusing species and what it is diffusing through. We use these timescales to better understand the timing of magmatic events relative to eruption, which improves hazard responses. Finally, the other timing method used within this group is textures of bubbles and crystals. Bubbles, and certain crystals, form in response to the loss of water from a magma during ascent to the surface. Thus, their textures, which includes number density and size distribution, track ascent processes immediately prior to eruption, which can be used to infer processes within the conduit that impact volcanic eruption style.
Experimental petrology
Igneous processes are dependent on many complex, interconnected variables that can be difficult to tease apart in a natural setting. Experimental petrology allows us to isolate variables in controlled environments to investigate their role in a variety of settings, including magma storage, magma ascent, and low temperature hydrothermal systems. For investigation of magma storage and ascent, we have externally-heated cold-seal pressure vessels that can be used to study phase equilibria at moderate pressure (25-250 MPa) and temperature (400 - 1000 ℃), which translates to shallow crustal magma storage. These experimental set ups are also capable of decompression, which allows for the investigation of ascent processes, such as bubble nucleation, growth, and coalescence, and crystal nucleation and growth. Knowledge of the concentration of base metals like Zn, Cu, and Pb in ancient hydrothermal fluids is fundamental to understanding how these fluids form base metal deposits. One way to obtain this concentration information is from partitioning theory. We are currently conducting laboratory experiments in which we precipitate dolomite from synthetic sedimentary brines in reaction vessels at temperatures and pressures typical of sediment-hosted ore deposit formation. The partition coefficients that we are finding can be used to determine the Zn, Cu, and Pb concentrations in ancient brines by measuring the Zn, Cu, and Pb concentrations in dolomite precipitated by those brines.
Subsurface fluid storage
The transition to a greener economy can be aided by effectively injecting and storing fluids in the subsurface. Capturing CO2 from point sources like electric power plants and injecting it into the subsurface can help reduce the accumulation of CO2 in the atmosphere. Hydrogen can be injected into and temporarily stored in the subsurface until times when intermittent energy sources like wind and solar are not able to meet energy demand. Petroleum reservoirs and saline aquifers can be favorable subsurface storage sites for CO2 and hydrogen. We use numerical models to simulate the behavior of CO2 and hydrogen in the subsurface, including how far these fluids flow from injection wells, how efficiently they can be extracted from production wells, how easily they can leak into overlying geologic formations, and how they react with rocks and other fluids in the subsurface.
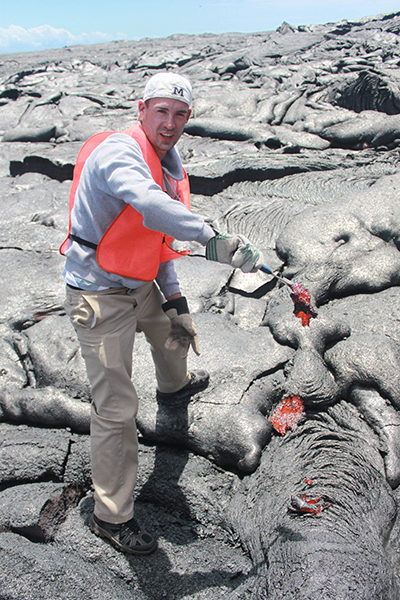